Application of anti-inflammatory treatment in two different ovine Acute Respiratory Distress Syndrome injury models: a preclinical randomized intervention study - Scientific Reports
Whilst the presence of 2 subphenotypes among the heterogenous Acute Respiratory Distress Syndrome (ARDS) population is becoming clinically accepted, subphenotype-specific treatment efficacy has yet to be prospectively tested. We investigated anti-inflammatory treatment in different ARDS models in sheep, previously shown similarities to human ARDS subphenotypes, in a preclinical, randomized, blinded study. Thirty anesthetized sheep were studied up to 48 h and randomized into: (a) OA: oleic acid (n = 15) and (b) OA-LPS: oleic acid and subsequent lipopolysaccharide (n = 15) to achieve a PaO2/FiO2 ratio of < 150 mmHg. Then, animals were randomly allocated to receive treatment with methylprednisolone or erythromycin or none. Assessed outcomes were oxygenation, pulmonary mechanics, hemodynamics and survival. All animals reached ARDS. Treatment with methylprednisolone, but not erythromycin, provided the highest therapeutic benefit in Ph2 animals, leading to a significant increase in PaO2/FiO2 ratio by reducing pulmonary edema, dead space ventilation and shunt fraction. Animals treated with methylprednisolone displayed a higher survival up to 48 h than all others. In animals treated with erythromycin, there was no treatment benefit regarding assessed physiological parameters and survival in both phenotypes. Treatment with methylprednisolone improves oxygenation and survival, more so in ovine phenotype 2 which resembles the human hyperinflammatory subphenotype. A preclinical randomized intervention study conducted at the Queensland University of Technology (QUT) Medical Engineering Facility (MERF) in Brisbane was approved by QUT Office of Research Ethics and Integrity (No 18-606). The study involved 30 animals randomized to the two injury methods: oleic acid (OA, n = 15) or oLEic acid and lipopolysaccharides (OA-LPS), and subjects were further randomized to receive treatment either with methylprednisolone (pred) OA-pred; each 5 per group) orerythromycin (ery). All sheep were approved for use by the facility veterinarian after a comprehensive health check and full blood count. Initial ventilation settings included tidal volumes of 8 ml/kg, a PEEP of 5 cmH 0, a respiratory rate of 12–16 breaths/min, and an arterial saturation of > 94% and normocapnia, defined by an end-tidal carbon dioxide (EtCO) of 35–45 mmHg.
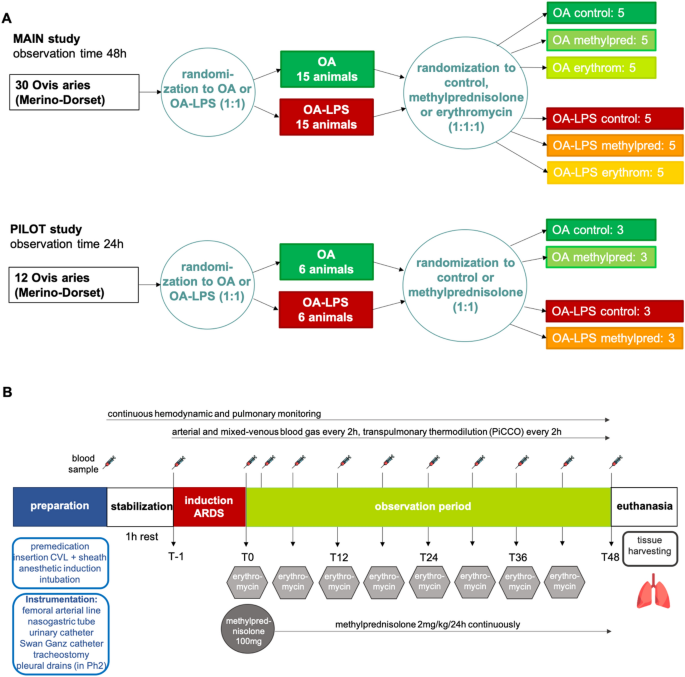
Published : 2 years ago by Carmen, Beijing, Wilson, The Prince Charles Hospital, Center for Cardiac Intensive Care, Silver, Wildi, Switzerland, John, Passmore, Fraser, Suen, Basel, San Martino Policlinico Hospital, Xiaomeng, University of Basel, Li Bassi, Jacky, Gatton, Integrated Diagnostics, Abbate, Department of Anaesthesia, Livingstone, Italy, Cardiovascular Surgery, The University of Queensland, Korea University, Gabriella, Colombo, Genoa, Katrina, Liu, People’s Republic of China, Karin, Galway University Hospitals, Critical Care, Ireland, College of Medicine, Sato, Galway, See Hoe, Wang, Margaret, Louise E., St Andrews War Memorial, Anesthesiology, Gianluigi, Fondazione IRCCS Ca’ Granda Ospedale Maggiore Policlinico, Christian, Samantha, Keibun, Pelosi, School of Veterinary Science, Jae-Seung, Neurosciences, The Wesley Intensive Care Units, Noriko, Ki, Queensland University of Technology, Sebastiano Maria, Kei, Emily, Bouquet, University of Galway, Kieran, Heinsar, Critical Care Research Group, University of Genoa, Laffey, Milan, Brisbane, Intensive Care Medicine, Hyslop, Capital Medical University, Ainola, Chiara, Palmieri, Department of Thoracic, Republic of Korea, Beijing Anzhen Hospital, Seoul, Australia, Paolo, Mueller, Department of Surgical Sciences, University Hospital Basel, Cardiovascular Research Institute Basel, Mahé, IRCCS for Oncology, Jung, Uniting Care Hospitals in Health
Animal studies were conducted at the Queensland University of Technology (QUT) Medical Engineering Facility (MERF) in Brisbane. Animal ethics was approved by QUT Office of Research Ethics and Integrity (No 18-606). All experiments were performed in accordance with the Australian Code of Practice for the Care and Use of Animals for Scientific Purposes and the Animal care and Protection Act 2001 (QLD) and complied with the ARRIVE Guidelines.
This was a single-blinded, randomized, controlled preclinical trial in an ovine model (Fig. 1A). A total of 30 animals were randomized and allocated to the two injury methods: oleic acid (OA, n = 15) or oleic acid and lipopolysaccharides (OA-LPS, n = 15). For each injury model, subjects were further randomized to receive treatment either with methylprednisolone (pred: OA-LPS-pred, OA-pred; each 5 per group) or erythromycin (ery: OA-LPS-ery, OA-ery; each 5 per group) or they were randomized to the control group (ctr: OA-LPS-ctr and OA-ctr; each 5 per group). A pilot study consisting of 12 animals was conducted to confirm safety, adherence to protocol and efficacy assumptions in relation to treatment (Fig. 1A): animals were randomized to either pred or ctr per each phenotype, resulting in 3 animals per group. Randomization was performed using a random number generator.
All sheep were approved for use by the facility veterinarian after a comprehensive health check and full blood count. Prior to experimentation, all animals were fasted overnight with free access to drinking water. A four-lumen central venous catheter (CVL; Arrow Int., Reading, USA) and a venous sheath (Edwards Lifesciences, Irvine, USA) were inserted in the jugular veins via Seldinger technique and sutured in place. Cefazolin 1 g intravenously (IV) was administered for antibiotic prophylaxis. For induction of anesthesia, midazolam (0.5 mg/kg) and propofol (3–4 mg/kg) were administered IV. A cuffed endotracheal tube size 9.0 internal diameter (Mallinckrodt, USA) was inserted into the airway, and mechanical ventilation (Galileo 5, Hamilton Medical, Switzerland) was commenced after placing the animal on the operative table in supine position. Initial ventilation settings consisted of tidal volumes of 8 ml/kg, a PEEP of 5 cmH 0, a respiratory rate of 12–16 breaths/min and an FiO of 30–50%; settings were adapted to maintain an arterial saturation of > 94% and normocapnia, defined by an end-tidal carbon dioxide (EtCO ) of 35–45 mmHg. General anesthesia was maintained and titrated to an appropriate level with continuous infusion of midazolam 0.5–0.8 mg/kg/h, fentanyl 5–20 µg/kg/h and ketamine 2.5–7.5 mg/kg/h. Basic monitoring consisted of a pulse oximeter probe attached to the tongue, a 5-lead electrocardiogram and continuous waveform capnography. A PiCCO catheter (PULSION Medical Systems, Getinge, Gothenburg, Sweden) was inserted into the femoral artery for advanced hemodynamic monitoring. A pulmonary artery catheter (Swan-Ganz CCOmbo, Edwards Lifescience, Irvine, USA) was inserted via the internal jugular sheath to monitor pulmonary artery pressure, mixed-venous saturation and core body temperature. In addition, a 14F nasogastric tube was placed and left on free drainage, and a 12F urinary catheter was inserted with closed collection. To facilitate access to the airways for bronchoscopy and decrease dead space ventilation, a surgical tracheotomy with insertion of a cuffed endotracheal tube (Size 9–10, Portex) was performed. During instrumentation, a crystalloid bolus (Hartmanns) of 250 ml was delivered to compensate for overnight fasting, then the fluids infusion rate was maintained at 1 ml/kg/h and modified as according to the hemodynamic condition. After instrumentation, the animal was positioned in prone position and rested for one hour prior to commencement of induction of ARDS (Fig. 1B).
Using the official ARDS definition for experimental models18,19, animals randomized to OA received sequential IV administration of oleic acid (0.89 g/ml; Sigma-Aldrich, Australia) in subsequent 0.03 ml/kg doses to ultimately achieve a PaO /FiO < 150, as assessed via arterial blood gases test 15 min after OA infusion20. OA-LPS group received administration of OA, then after fulfilling ARDS criteria, 0.5 µg/kg of lipopolysaccharide (LPS: E. coli O55:B5, Sigma-Aldrich, Australia), dissolved in 50 ml of normal saline, was infused over 1 h.
Animals randomized to treatment with methylprednisolone received a bolus of 100 mg IV at confirmation of ARDS diagnosis (T0) and a continuous infusion of 2 mg/kg/24 h over the course of the study (Fig. 1B). Animals randomized to treatment with erythromycin received a bolus of 100 mg IV every 6 h, starting at T0. Investigators involved in the clinical management of the study subjects were blinded to treatment allocation: all animals received two 10 ml boluses at T0 (one containing methylprednisolone 100 mg or normal saline (NS), and another containing erythromycin 100 mg or NS), followed by a continuous infusion of 2 ml/h (containing methylprednisolone 2 mg/kg/24 h or NS) and a 10 ml bolus every 6 h (containing erythromycin 100 mg or NS).
Arterial blood gas analysis was performed every 2 h and whenever clinically indicated to assess blood gases, glucose and electrolytes. Mixed-venous blood gas analysis and transpulmonary thermodilution using Pulse Contour Cardiac Output (PiCCO) technology was performed every 2 h (Fig. 1B). Intra-experimental monitoring and data recording are described in the Supplemental Methods.
To minimize potential confounders, strict protocols for hemodynamic management (Supplemental Fig. S1), ventilation strategy (Supplemental Fig. S2) and procedures were applied.
Animals were euthanized with 163 mg/kg of pentobarbital and organs were retrieved for tissue harvesting at the end of the study, 48 h after development of ARDS, or when animals reached the following criteria: (a) mean arterial blood pressure (MAP) persistently below 40 mmHg despite optimized support; and (b) arterial pH below 7.0 because of respiratory, metabolic or mixed acidosis and exhausted compensation strategies.
The primary outcome was oxygenation (PaO /FiO ratio (PF ratio) and Oxygenation Index (OI)) throughout the 48 h assessment period among injury types and their respective treatment. As secondary outcomes, we investigated the effects of study treatment on lung edema (extravascular lung water index (EVLWI)), hemodynamic parameters, pulmonary mechanics, and survival in the respective lung injury.
Blood samples for full blood count (Mindray Hematology analyzer BC 5000, China) and biochemistry (IDEXX laboratories Brisbane, Australia) were collected at baseline and every 12 h following T0. Postmortem collected lung tissue samples were prepared for histopathology. Quantification of pulmonary edema was determined by measuring the wet-to-dry-weight of post-mortem left and right upper and lower lung lobe: a tissue sample of 2 cm diameter and 2 cm thickness was excised and lung weight measured immediately. Dry weight was determined by placing the lung tissue in an oven at 65 °C for several days, weighing the tissue every day until the weight stopped decreasing. The wet-to-dry weight ratio was calculated by dividing the wet by the dry weight.
Arterial blood samples were collected in EDTA blood tubes, processed to plasma and stored at − 80 °C until analysis. Plasma concentration of inflammatory cytokines (e.g. interleukin (IL) -6, -8, -10, -1β) in serum were quantified by in-house ELISA’s21.
At study end, lung tissues were taken for histological assessment (Suppl. methods). Slides were examined by blinded, qualified veterinary pathologist. The lung injury score (LIS) was assessed as recommended by the ATS for experimental ARDS in animal models18,19: it scores neutrophils in the alveolar space (A) and in the interstitial space (B), hyaline membranes (C), proteinaceous debris filling the airspace (D) and alveolar septal thickening (E). Every item is given a score between 0 to 2, then the LIS is calculated by: ((20 × A) + (14 × B) + (7 × C) + (7 × D) + (2 × E))/number of fields × 100. This results in a score between 0 (no injury) and 1 (severe lung injury).
Twenty random high-power fields (400 × total magnification) were scored per section and the LIS was calculated per animal (mean ± SD). For the scoring, at least 50% of each field had to be filled by lung alveoli: fields consisting predominantly of the lumen of large airways or vessels were rejected. Septal thickness was not evaluated in alveolar septa directly adjacent to a blood vessel or airway (normally thickened by the collagen present in the peribroncho-vascular bundle). In addition, the following parameters were evaluated: (a) percentage of section effaced by necrosis (score/field: 0 = none or less than 10%; 1 = 10–50%; 2 = > 50%) and (b) number of thrombi within blood vessels (score/field: 0 = none; 1 = 1 thrombus; 2 = > 1 thrombus). Bacterial colonies as observed in necrotic areas or admixed with the neutrophilic aggregates and alveolar hemorrhage were marked as present or not within one animal and reported as percentage per injury type and the respective treatment group.
For the power calculation, an F-test for correction to 6 treatment groups was applied (power set at 80%, level of α at 5%, 2-tailed), assuming a treatment effect in terms of an improvement in PaO /FiO ratio of 80 mmHg and a standard deviation (SD) of 40 mmHg22 over the course of the study. Conservatively assuming a Cohens d of 1.0 (medium effect size), this resulted in a total sample size of 28 animals, therefore 5 animals per group.
Categorical variables are reported as numbers and percentages, continuous data as mean ± SD or median and interquartile range (IQR), for normally or not-normally distributed parameters, respectively. Comparisons between groups were made using Kruskal–Wallis or Mann–Whitney U test and x2 test as appropriate.
Linear-mixed effects models (LMM)23 were constructed to assess the impact of parameters of oxygenation, hemodynamics, metabolic situation and respiratory mechanics over time among injury types and their treatment. Distribution of data was assessed with QQ and residual plots. Control animals were defined as the reference level, time was specified as a continuous fixed effect and the interaction between treatment and time was included. A random effect was defined for each individual to account for within-subject correlation from repeated measurements over time. Fixed effects were reported as estimates with 95% confidence intervals (CI) and p-values were estimated using Sattherwaite’s method.
The pairwise comparison of injury types (2 groups) over time was performed using averaging over time and injury type, the Kenward-Roger method was applied for the degrees of freedom.
A shared parameter joint model24 for assessment of longitudinal and time-to-event data (death) and the associated treatment effect was estimated under a Bayesian framework. The model uses observed longitudinal measurement (trajectories) to determine the posterior probability for event (death) and treatment effect for each of the assessed parameter. Data were reported as hazard ratio (HR) and 95% credible intervals.
All statistical analyses were performed with R Version 4.0.5 (R Foundation for Statistical Computing, Vienna, Austria). The minimal reproducible code for the LMM and Bayesian model is reported in the Supplemental Methods.